
Neutrinos are weakly interacting particles that are able to travel undeflected through the cosmos. The IceCube Neutrino Observatory and the KM3NeT Astroparticle Research with Cosmics in the Abyss (ARCA) telescope (still under construction) are cubic-kilometer-scale neutrino telescopes that search for the sources of these astrophysical neutrinos in hopes of uncovering the origin of ultra-high-energy cosmic rays (UHECRs).
The highest-energy astrophysical neutrinos, having energies beyond 1 PeV, require particles with an incredible amount of energy in order to be produced. One component, called cosmogenic neutrinos, are expected to be produced when the highest-energy cosmic rays interact with the cosmic microwave background, producing charged particles that decay to neutrinos. Named after the theoretical upper limit of cosmic-ray energies (the Greisen–Zatsepin–Kuzmin or GZK limit), these extremely high-energy neutrinos make up the GZK neutrino flux. Even though scientists know this flux exists, they have yet to detect it.
For the first time, researchers at the Wisconsin IceCube Particle Astrophysics Center (WIPAC) of the University of Wisconsin–Madison (UW–Madison) combined 10 years of IceCube data with UHECR data from the Pierre Auger Observatory and data from the recent KM3Net event—possibly the highest energy neutrino discovered—to explore a possible source common to high-energy neutrinos and UHECRs. They show that the model is able to describe the combined data across these three observatories and hints at an emerging population of neutrinos produced by UHECRs.
The researchers explored two possible scenarios for the KM3NeT neutrino observation’s energy: 100 PeV and 1 EeV. They tested the hypothesis that, in either case, an astrophysical model for the neutrino flux could explain both the highest-energy neutrino events observed by IceCube and KM3NeT while also explaining the cosmic-ray data from the Pierre Auger Observatory.
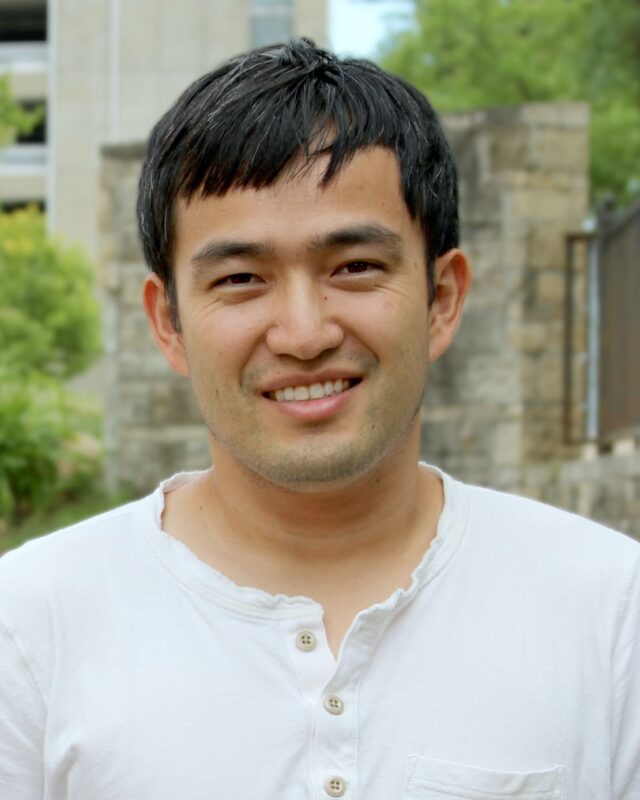
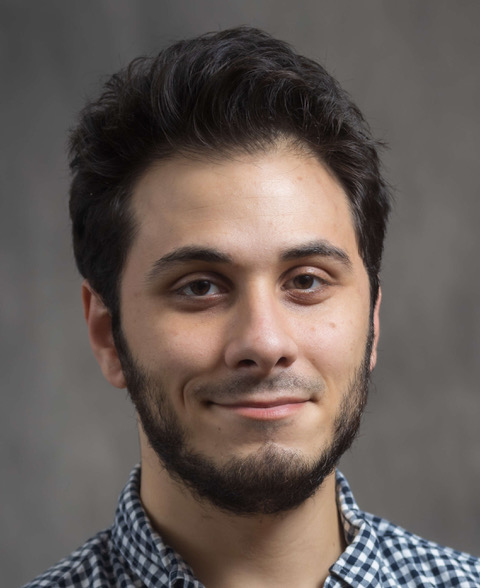
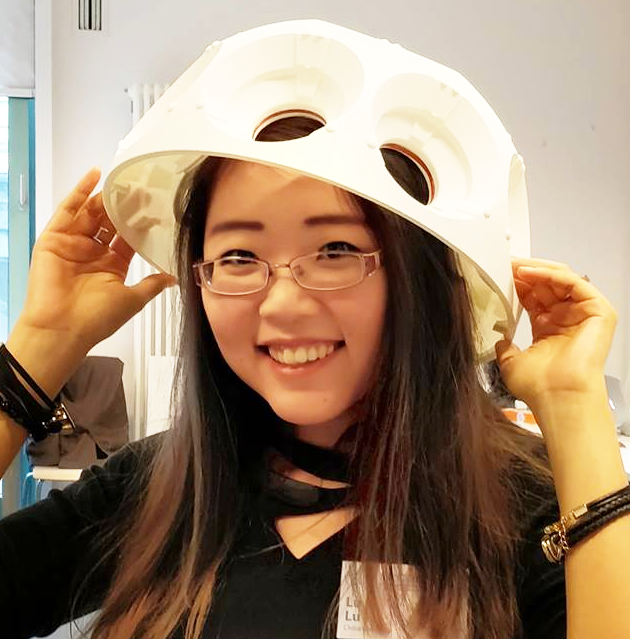
“This is something that hasn’t been done before, and it allows us to test if neutrinos and UHECRs have a common origin,” says Tianlu Yuan, an assistant scientist at WIPAC who contributed to the analysis. “The KM3NeT event is possibly the highest-energy neutrino and, potentially, the first GZK neutrino discovered, and so by combining that result with IceCube data, we wanted to make a model-independent measurement of the diffuse neutrino flux at the highest energies.”
The model assumed that both data sets are produced by the most extreme accelerators in the universe, namely cosmic rays that are accelerated and interact with their sources before producing neutrinos that travel to the Earth. To test their hypothesis, the researchers fitted the global neutrino dataset of the highest-energy neutrinos (> 5 PeV) from both IceCube and KM3NeT simultaneously with the cosmic-ray spectrum and (chemical) composition data from the Pierre Auger Observatory.
“We compared the predicted neutrino fluxes from this model to a single power-law model, a common expectation in astrophysics that does not assume any connection to cosmic rays,” says Marco Muzio, a John Bachall postdoctoral fellow at WIPAC and corresponding author on the study. “In both cases, either a 100 PeV or 1 EeV neutrino, we found that the astrophysical model is able to give a slightly better explanation of the highest-energy neutrino data than a single power-law model, while also explaining the cosmic-ray data, providing a tantalizing hint at the common origin of both of these cosmic messengers.”
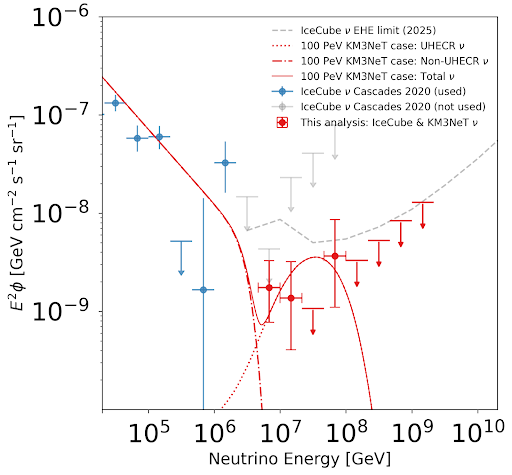
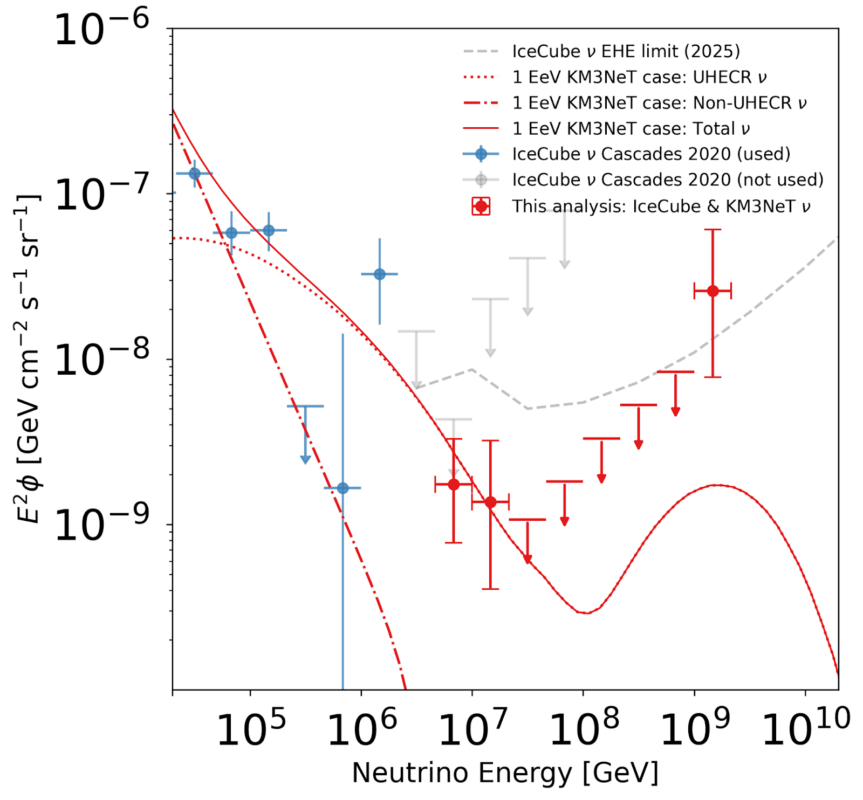
neutrino flux for the model that best describes the combined neutrino and cosmic ray data. The right panel is the same but assumes an energy of 1 EeV. The IceCube measured flux is shown in solid blue and gray, with the lower energy values (blue) included in the fits. Differential upper limits (90% CL) are shown as the dashed gray line. A model-independent flux obtained by fitting independent normalization parameters on piecewise segments of an E−2 spectrum to the high-energy neutrino data is shown in red.
Muzio notes, however, that the implications depend on the particular energy of the KM3NeT neutrino. In the 100 PeV case, the KM3NeT event, along with the highest-energy event from IceCube, would represent the emergence of a population of neutrinos produced by UHECR interactions with photons inside their sources.
For the 1 EeV case, it would suggest the first cosmogenic neutrino produced by cosmic-ray protons beyond 50 EeV. This observation would provide compelling evidence for a cosmic-ray proton component at the highest energies that is complementary to the observations from other ultra-high-energy observatories. Neutrinos at EeV energies along with the protons that produce them could allow scientists to directly identify sources of UHECRs since they would both point back towards their sources.
“If the latest high-energy event observed by KM3NeT is indeed a neutrino, it remains unclear whether it was produced directly at an UHECR source or during propagation. However, both scenarios remain consistent with current experimental data,” says Lu Lu, an assistant professor of physics at UW–Madison and part of the analysis team. “At present, IceCube is utilizing transformer-based neural networks to classify and reconstruct high-energy events. These AI-driven techniques are enhancing our ability to analyze the most energetic events and will also improve the accuracy of event reconstructions, which is especially important for the time-domain multimessenger community.”
Given that current kilometer-scale neutrino observatories have detected only a single event around 100 PeV over more than a decade, the statistical uncertainties are still very high, calling for larger detectors that can gather more data. For IceCube, that is the proposed IceCube-Gen2, which will be about ten times larger and more sensitive to ultra-high-energy neutrinos thanks to a radio component. With these improvements, physicists may soon make a new discovery in neutrino astronomy.
+info “Emergence of a neutrino flux above 5 PeV and implications for ultrahigh energy cosmic rays,” Marco S. Muzio, Tianlu Yuan and Lu Lu, arxiv.org/abs/2502.06944