Extremely energetic charged particles called cosmic rays are accelerated by violent cosmic objects before raining down on Earth from all directions. Protons and heavier nuclei make up these cosmic rays, which can then collide with other particles in the atmosphere to produce secondary particles that cascade into so-called “air showers.”
For more than a century, scientists have tried to uncover the origins of cosmic rays by investigating their properties. Anisotropy, the property of having characteristics that change depending on the observed direction, can be measured in cosmic rays to better understand them and the origins of their sources.
Characteristics such as the energy spectrum and arrival direction of cosmic rays are influenced by their propagation through the interstellar medium and the distribution of their sources. Experiments like the IceCube Neutrino Observatory, a gigaton-scale neutrino detector at the South Pole, capture high-energy muons produced by cosmic-ray particles that collide with the Earth’s atmosphere. Since these muons share the direction of the cosmic ray from which it came to within a fraction of a degree, they can be used to measure the cosmic-ray arrival direction distribution at energies of 10 TeV and above.
In a new study submitted to The Astrophysical Journal, the IceCube Collaboration presents the most accurate measurement of the cosmic-ray arrival direction distribution from 10 TeV to several PeV using 12 years of IceCube data, equating to almost a trillion cosmic-ray events. This dataset provides an up-to-date cosmic-ray arrival direction distribution in the Southern Hemisphere with unprecedented statistical accuracy throughout the eleven-year solar cycle.
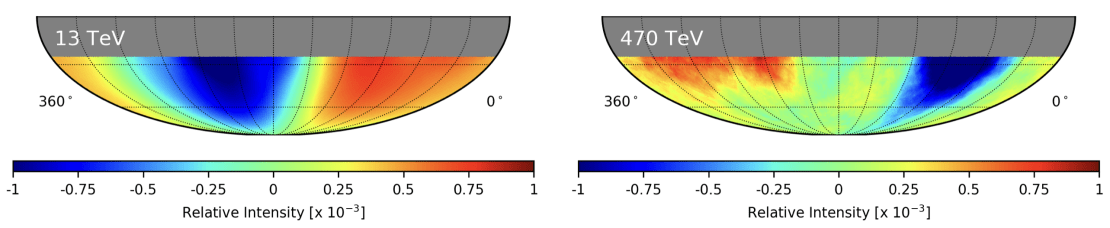
Cosmic rays are almost isotropic in that their flux is approximately the same regardless of direction. The variation of the cosmic-ray flux changes only by about 0.1% across the sky, with such a small anisotropy requiring specialized methods to ensure accurate measurements of the deviation from isotropy.
In order to measure the deviation, a perfectly isotropic flux of cosmic rays, or reference arrival direction map, was first determined by the collaborators. They calculated the reference map by changing each event’s arrival time and picking a random value within the 24-hour day duration. By doing this, the measured arrival direction of the cosmic-ray particle is scrambled, and the anisotropy is washed out, with only the angular distribution of the experiment’s response accurately maintained in the data.
After determining the reference map, the collaborators used that and IceCube data to calculate the relative intensity or the relative difference between the data arrival map (with anisotropy) and the reference map (without anisotropy). Improved simulation data was also used to estimate the energy of the cosmic rays and split the data sample into different energy ranges, with median energies from about 10 TeV to 5 PeV.
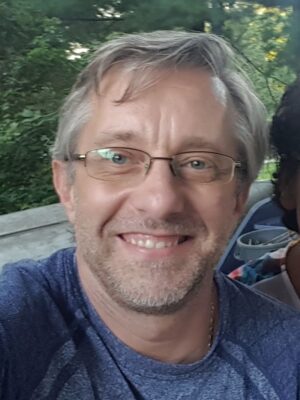

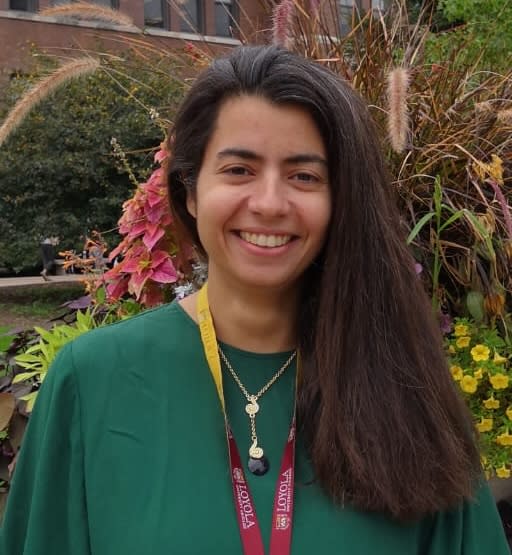
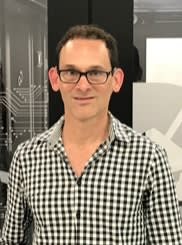
“However, these simulations are not accurate because the uncertainties in the particle collisions are significantly larger than the 0.1% anisotropy’s amplitude,” explains Paolo Desiati, an assistant research professor at the Wisconsin IceCube Particle Astrophysics Center (WIPAC) at the University of Wisconsin–Madison (UW–Madison) and co-lead on the study. “For this reason, we use the data themselves to calculate the reference maps.”
The study was also co-led by Mercer University associate professor Frank McNally, Loyola University Chicago assistant professor Rasha Abbasi, and WIPAC simulation coordinator Juan Carlos Díaz Vélez. It also involved undergraduate contributions from Timothy Aguado, Katherine Gruchot, William Hayes, Andrew Moy (Loyola), Christina Cochling, Alexis Hardy, Emily Schmidt, Alexander Simmons, Andrew Thorpe (Mercer), and Hannah Woodward (UW-REU).
“This analysis provided a unique opportunity to involve several undergraduate students in research early in their academic careers,” says Abbasi. “Including physics undergraduates in research is crucial for their education, as hands-on experience fosters critical thinking, problem-solving skills, and a deeper understanding of their field. Their fresh perspectives and contributions also enhanced the research process, leading to, in some cases, innovative ideas and insights.”
“When compiling relative intensity maps at different cosmic-ray energies, we were able to visualize not only the shape of their anisotropy, but also how this shape changes with energy,” says Desiati. “IceCube is the only experiment located in the Southern Hemisphere that is performing such measurements in the TeV-PeV energy range. This means that IceCube makes it possible to provide a complete view of the arrival direction of the cosmic rays, in combination with the Northern Hemisphere observatories.” (See the combined HAWC and IceCube observation: https://doi.org/10.3847/1538-4357/aaf5cc.) The dataset used for this study is also publicly available.
An outstanding question is whether the anisotropy changes with time across the eleven-year solar cycle. Solar cycles can affect the entire heliosphere—the magnetic bubble surrounding the solar system formed by the interaction between the expanding solar wind and the interstellar medium—which in turn can affect the arrival direction of the cosmic rays.
“If this is true, we will be able to demonstrate the dynamic nature of cosmic-ray observations in the TeV energy range, as well. And, perhaps, increase our understanding of the heliosphere as a whole,” says Díaz Vélez.
In addition, the data collected by IceCube will also allow researchers to measure whether the cosmic-ray anisotropy is associated with anomalies in their energy distribution. Even though the cosmic-ray energy distribution, or spectrum, is measured with great precision by many experiments, there is still a question of whether the propagation of the cosmic-ray particle produces anisotropy in energy associated with anisotropy in the arrival direction. Even though this has been observed in the ultra-high-energy range, it has not been observed at lower energies.
“The reason may be that directional scrambling due to propagation may easily wash out energy anomalies,” says McNally. “Or, if such energy anomalies exist, they may be too small to be observed. Now, we have a chance to measure this.”
+ info “Observation of Cosmic-Ray Anisotropy in the Southern Hemisphere with Twelve Years of Data Collected by the IceCube Neutrino Observatory,” IceCube Collaboration: R. Abbasi et al. Submitted to The Astrophysical Journal. arxiv.org/abs/2412.05046